Build a better bus
5 April 2022
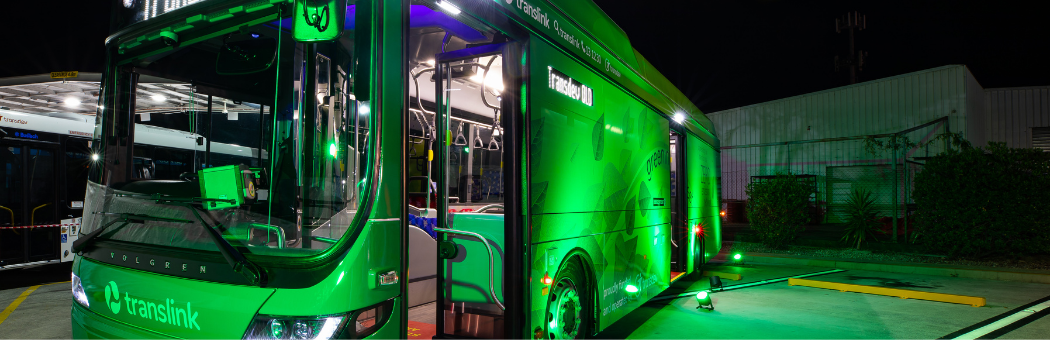
Many consider the new Victorian distance tax on electric vehicles an outrage that will hinder the adoption of this important technology. On the other hand, the government is doing other things to accelerate the adoption of emissions-free transport.
One example is the $2.3 billion contract awarded to national bus operator, Kinetic last year to bring electric buses into service across the Metropolitan Bus Franchise. The contract runs until 2030 and will fund the introduction of hundreds of plug-in battery and hybrid buses to routes currently served by diesel-powered units. Thirty-six of these will be in service by 2025. Importantly, the bus bodies will be built locally by Volgren at its Dandenong South plant.
Other bus companies are also adopting electric buses and Melbourne operator, Ventura is currently trialling a Volgren bodied battery-electric bus out of its Ivanhoe depot. This, and other programmes around the country, all constitute the thin end of the wedge. What’s more, the opportunity for expansion in this transport industry segment is so great that we’re going to be at the thin end of the wedge for years to come.
Buses are subject to stresses similar to those in cars, only more so. Because they’re so long Z-axis bending, lateral bending and torsional twisting are all more pronounced than they are in cars. Monocoque construction isn’t an option for something as long as a bus with flat sides and a long roof line. This means a body built on a steel chassis is necessary, as with virtually all larger commercial vehicles.
Volgren, as a bus bodybuilder, works in partnership with the main global chassis OEMs. Volgren imports the chassis used in its electric buses. These are specialised units from BYD designed and built around electric hub motors and support components for them like inverters, controllers and batteries. The batteries in these buses are mounted on the roof, but more on batteries shortly. Hub motors aren’t really suitable for cars because they contribute to unsprung weight, which is highly detrimental to handling. This is not such an issue in buses because they aren’t subjected to high-speed cornering and other demands that require the nimble responses of a car. So, setting the electric motors in the wheels is an excellent choice for a bus. Doing so eliminates the need for mechanical transfer hardware like drive shafts and differentials resulting in less weight and reduced servicing requirements.
Weight is an important consideration in any vehicle but particularly so in battery electric vehicles like these buses. Less weight means greater range from each charge. In turn, this means longer runs that can be a greater distance from charging stations at company depots. Given buses make regular stops at predicted locations, there are some quick-charge options appearing in Europe that are of interest. At some stops, roof located arms (pantographs) are extended to couple with quick charge outlets that can push a significant charge into battery packs to extend range. The first of these flash charging pantographs in Australia is being constructed right now as part of the $1.244 billion Brisbane Metro Project. Swiss company, HESS won the contract to deliver 60 bi-articualted, 25-metre electric vehicles and work with charging partner Hitachi.
HESS also offers a unique technology for bus construction called Co-Bolt. Volgren, who are responsible for two out of every three route bus bodies in Melbourne, has used this technology in the body construction since 1977. It’s a system of aluminium C-formed extrusions joined by cast corner pieces and clamping plates held in place with bolts. Importantly, welding is not required with the Co-Bolt system. Bolts may not seem like the ideal joiners for vehicles due to NVH concerns but the bolts in this system are fitted and held firmly in place using a two-part adhesive that prevents them from coming loose due to the rigours of operating stresses. Despite this, they are readily removable if necessary. No welds also means no cracks.
The exterior panels of buses built using the Co-Bolt system couple with the frame fairly simply. They are made to be easily removable in case of damage. Removing and replacing a plain side panel is said to take only a couple of minutes and hinged access panel replacement takes just five minutes according to HESS. The Co-Bolt system has been extensively tested and used to construct more than 20,000 bus bodies worldwide. As you’d expect, crash, roll-over and stress tests are among those that various authorities have imposed on the system. In fact, aluminium demonstrates very good energy absorption in crash tests and doesn’t suffer from corrosion like steel. Also, in keeping with environmental concerns, aluminium is completely recyclable.
These days mention of anything battery electric brings to mind Li-ion batteries. Li-ion is a generic term covering batteries that use a number of different materials. Volgren uses Lithium Iron Phosphate (LiFePO4 or abbreviated - LFP) types. These are becoming increasingly popular. LFP is a type of Li-ion battery but with its own particular chemistry.
In an LFP battery, the positive electrode contains a thin layer of LiFePO4 deposited on aluminium foil. The negative electrode is made from graphite deposited on copper foil. The graphite is specifically engineered to receive lithium ions. These positive and negative plates are immersed in an organic solvent-based electrolyte that also contains a microporous sheet called a separator. The microstructure of the separator is such that it allows lithium ions to pass but blocks electrons. So, apart from the materials used, a LiFePO4 battery is broadly similar to any other battery.
Charging current releases lithium ions from the LiFePO4 into the electrolyte where it passes through the separator and into the graphite layer of the negative electrode. The electrons in the charging current flow into the negative electrode where they combine with the lithium ions. This process removes electrons that were associated with the lithium in the positive plate.
The cell is now charged. In this condition, the positive plate has become electronegative. That is, it attracts electrons but there are none to be had. They’re over in the graphite matrix at the negative electrode. As with any battery, when the positive and negative electrodes are connected via an external load, like a bus motor, the electrons flow back into the positive electrode. The lithium ions flow back to the positive plate through the electrolyte where they recombine with the electrons and iron phosphate to recreate LiFePO4 once again. This back and forth transfer of lithium ions gives rise to the term ‘rocking chair’ battery.
The composition of the electrodes and electrolyte in Li-ion batteries don’t undergo chemical changes during charge and discharge in the same way they do in, say, a lead/acid battery. This is one reason Li-ion batteries generally don’t have memory effects and why they have a longer service life than other types of cells. It’s important to note that the description above is highly simplified with many details omitted. There’s a great deal more going on in the electrolyte and at the separator.
More important here than all the details of the processes at work in a lithium iron phosphate cell are the overall characteristics of LiFePO4 cells. First, they have a lower charge density than other types of Li-ion batteries. That means a vehicle equipped with them has a shorter range than one powered by more common Li-ion cells. So, why does BYD use them? Well, LiFePO4 cells can be charged about twice as many times as other Li-ion batteries. That’s obviously an advantage for a bus that’s likely to run down to minimum voltage or close to it every day. At the moment these buses have a range of up to 300km per charge.
The oxygen in LiFePO4 is more tightly bound to the molecule than in other Li-ion chemistries. This means it doesn’t support the sort of self-sustaining combustion we’ve seen in some vehicles when the battery packs are damaged. It’s true the electrolyte in an LFP cell can still burn, but reducing the likelihood of a vigorous fire that’s difficult to extinguish because it’s fed by internal oxygen is an excellent idea for a bus full of people. Last, LiFePO4 cells don’t contain cobalt or manganese, which is excellent for the third world labourers who have to mine these metals under back breaking conditions. The materials used in BYD LiFePO4 batteries are also highly and cleanly recyclable, another win for the environment.
In most battery electric vehicles the battery forms part of the floor. However, no one wants to climb a staircase to get a seat on a bus so the lower the floor, the better. Placing the batteries on the roof of a bus helps keep the floor as low as possible. Now, it’s also important to keep the centre of gravity of a vehicle as low as possible for stability, yet putting a heavy bank of batteries on the roof contravenes this basic automotive design principle. Again, though, things are different with buses. They certainly go around corners and curves but at speeds and turn radii that create far less roll.
As mentioned above, the BYD chassis Volgren uses for these buses has two AC synchronous hub motors at the rear wheels. Although batteries supply DC electricity, virtually all modern electric vehicles are powered by AC motors. This is because the speed of AC motors can be very finely controlled by adjusting the frequency of the AC power fed to them.
Like common industrial induction motors, the rotor in an AC synchronous motor is driven by rotating electromagnetic fields in the stator, or at least that’s how it’s commonly described. The fields don’t really ‘rotate’. Rather, an AC motor has a number of field windings forming poles in the stator. These are switched on and off sequentially in the right order and with suitable timing to create the so-called rotating field. The rotor holds a number of permanent, extremely strong rare earth magnets that are attracted to the fields in the stator and the rotor rotates to keep up with them.
We mentioned switching. At its simplest, that’s what a motor controller is, a box of cleverly arranged switches that energise sets of windings in the stator at just the right time. They create simulated AC output by pulse width modulation. At the beginning of the simulated sine wave they switch on and off very quickly. As they stay on for longer they simulate the increasing profile of a sine wave and finally at the peak of the simulated sine wave they stay on for the maximum amount of time. Then, they reduce in duration until the simulated sine wave reaches zero. At that point another set of switches takes over to emulate the negative, or reverse, part of the simulated sine wave. Thus, one AC cycle.
As the sine wave is digitally simulated its profile can be tailored to the exact speed and torque requirements needed by the motor(s) to match moment by moment operating demands. Digitally controlled systems like this are ideal for incorporating programmable logic input. These days we’re seeing the introduction of interlinked AI (Artificial Intelligence) systems that can read instantaneous conditions and deliver tailored adjustment and control. More importantly, such embedded AI can learn and make predictive assessments of how to deliver power for the most effective performance and in a way that will maximise utilisation of available battery capacity.
Again, this is just the most basic description of how motors and controllers work. The most important factor in this story is what’s going on inside the bus from the passengers’ and driver’s perspectives. Specifically, not much. Just comfort, smoothness and a considerately quieter ride.
As featured in Australasian Automotive April 2022.
518